We use cookies on this website. Cookies help us deliver the best experience on our website. Read about cookies.
-
- Education
- Education
- Programmes and courses
- Applications and admissions
- Tuition fees
- Scholarships
- Exchange studies at Malmö University
- Study Guidance
-
- After admission
- After admission
- Moving to Malmö
- Pre-orientation
- Arrival guide
-
- About studies at Malmö University
- About studies at Malmö University
- Why choose Malmö University
- Understanding university studies
- Connect with our students
On the page -
- Research
- Research
-
- Doctoral studies
- Doctoral studies
- Doctoral courses
-
- Doctoral schools
- Doctoral schools
- Doctoral school: Education, Learning and Globalisation
- Doctoral school: The National Research School for Professionals in Social Services
- Doctoral school: Learning in Multicultural Societal Contexts
- Doctoral school: ComBine
- Doctoral school: Swedish National Graduate School in Science and Technology Education Research
- Doctoral school: Relevancing Mathematics and Science Education (RelMaS)
- Doctoral school: Sustainable Movement Education
- Doctoral school: Finding ways in a time of great future challenges (FinnFram)
- Doctoral school: Pedagogy and Vocational Skills
- Doctoral school: Culturally Empowering Education through Language and Literature
- Research subjects
-
- Research centres
- Research centres
- Biofilms Research Centre for Biointerfaces
- Citizen Health
- Imagining and Co-Creating Futures
- Institute for Urban Research
- Malmö Institute for Migration Studies
- Literacy and Inclusive Teaching
- Centre for Work Life Studies
- Sustainable Digitalisation Research Centre
- Centre for Sexology and Sexuality Studies
-
- Research publications
- Research publications
- Search publications
- Malmö University Press
- Research events
- Participate in a research study
- Coffee Break Quiz
On the page -
- Collaboration and Innovation
- Collaboration and Innovation
- Innovation
- Collaboration with students
-
- Collaborate with researchers
- Collaborate with researchers
- Labs and facilities
- Culture collaboration
- Support Malmö University
-
- Alumni & Friends
- Alumni & Friends
- Graduation and alumni network
On the page -
- About us
- About us
-
- Faculties and departments
- Faculties and departments
-
- Faculty of Culture and Society
- Faculty of Culture and Society
- Department of Urban Studies
- Department of Global Political Studies
- School of Arts and Communication
-
- Faculty of Education and Society
- Faculty of Education and Society
- Department of Childhood, Education and Society
- Department of Sports Sciences
- Department of Natural Science, Mathematics and Society
- Department of School Development and Leadership
- Department of Culture, Languages and Media
- Department of Society, Culture and Identity
-
- Faculty of Technology and Society
- Faculty of Technology and Society
- Department of Computer Science and Media Technology
- Department of Materials Science and Applied Mathematics
-
- Faculty of Odontology
- Faculty of Odontology
- Master's programmes in Dental Science
- University Dental Clinic
- Management and decision-making paths
-
- Vision, objectives and strategy 2025
- Vision, objectives and strategy 2025
- Global engagement
- Sustainability
- Widened recruitment and participation
- Quality assurance work at the University
-
- Malmö Academic Choir and Orchestra
- Malmö Academic Choir and Orchestra
- Student work – video pieces
-
- Annual Academic Celebration
- Annual Academic Celebration
- Academic traditions
- Meet our new professors
- The University in a troubled world
On the page
Materials Engineering
At Materials Science, we carry out fundamental research of industrial relevance. Our research is synergistic, encompassing modelling and experiments, and carried out on multiple spatial and temporal scales. We perform our studies at large-scale facilities (MAX IV, ESS, DESY,…) with significance for the whole society (reduced use of resources and minimized carbon emission).
Our multiscale research spans from electronic structure to continuum. On the electronic structure level, we employ several methods, including density functional theory and X-ray photoelectron spectroscopy. Molecular dynamics serve to unravel both atomic and nanoscale phenomena, whereby the corresponding experimental validation involves scanning tunnelling microscopy and small-angle scattering. On the mesoscale, phase field and diffraction methods are employed. Finally, on the continuum scale, finite element modelling and tomography are used. Our vision is to develop synergistic models for modern materials across multi scales to enable novel applications.
Microstructure evolution
Materials with appropriate properties have always been crucial for technological achievements. Understanding the microstructural evolution of materials is a key component in predicting their behaviour and tailoring their properties. We study how microstructures of different materials evolve during, e.g., mechanical loadings and heat treatments. A key component in our research is the development of computational material models and methods for data analysis. Current examples include novel ways of reconstructing strain fields from diffraction data and 4D (3D + time) segmentation methods for tracking the evolution of microstructural features.
Materials for catalysis and energy generation
Catalyst research focuses on the atomic-scale characterization of model materials with well-defined surface structures, using a combination of classical ultra-high vacuum methods as well as novel ambient-pressure methods based on synchrotron X-rays. The chemical and electronic properties of the materials for catalysis are probed. On the other side, the investigation of energy materials focuses not only on the properties related to energy conversion but also on interactions with the environment (e.g. soft matter). The vision is to identify new energy generation and storage mechanisms and to combine them in hybrid devices.
Environmental degradation of materials
The research is centred around investigating mechanisms associated with the degradation of materials that are subjected to high temperatures, mechanical load and/or generally harsh environments for the purpose of understanding the degradation over time, such that component lifetimes can be assessed. This ranges from corrosion and hydrogen embrittlement in engineering materials to radiation-induced degradation of reactor materials in fusion and fission reactors. Examples of ongoing work include modelling defect-induced hydride precipitation in metals, charting potential trap sites for hydrogen in high-strength steels and predicting radiation- and impurity-induced grain boundary embrittlement in nuclear reactor materials.
Researchers, publications and projects
Pedro Henrique Arantes Moya
Martin Fisk
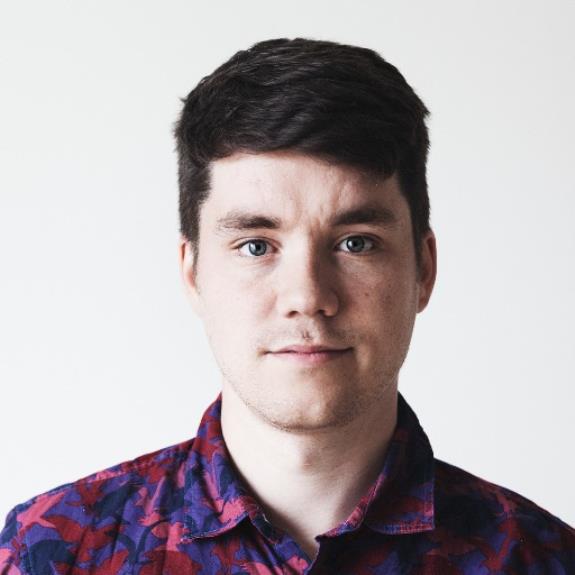
Johan Hektor
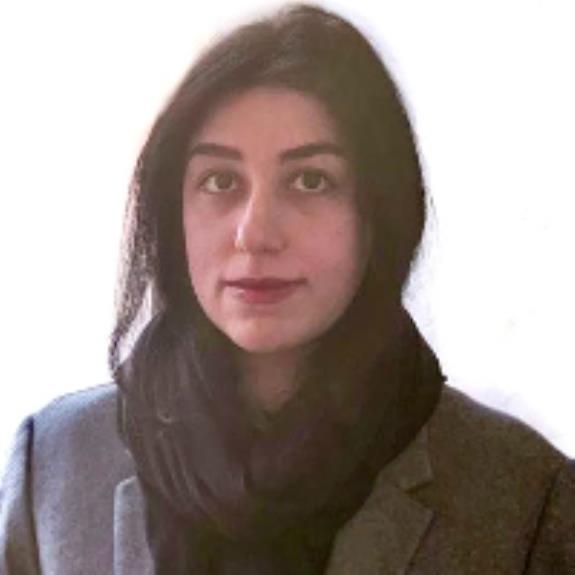
Sana Khayyamifar
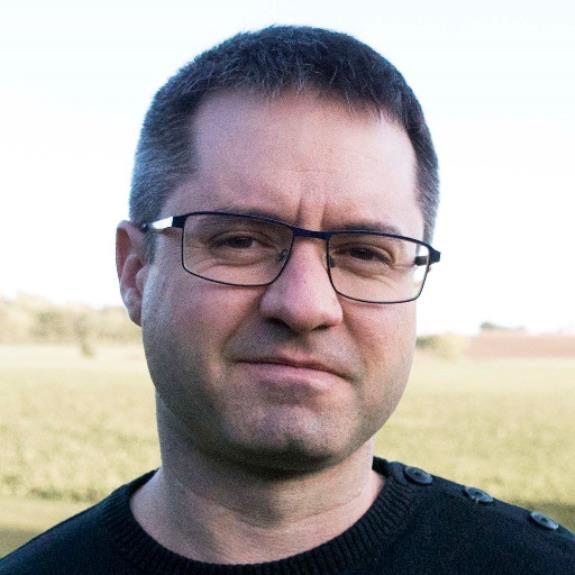
Lindsay Richard Merte
-
2025 | Article in journal
Combined in situ X-ray diffraction and tomography study on the evolution of the microstructure in 316L stainless steel foams during compression
Jenő Gubicza, Kadosa Nagy, Zoltán Hegedűs, Indrajeet Tambe, Péter Nagy, Johan Hektor, Chaehee Han, Heeman Choe
-
2025 | Article in journal
First-principles study of dynamic instability and phase transformation in the WRe and WOs sigma phase
Anders Vesti, Denis Music, Thomas Hammerschmidt, Mauro Palumbo, Pär A T Olsson
-
2025 | Article in journal
Data‐driven prediction of phase formation in graphene–metal systems based on phase diagram insights
Leilei Chen, Changheng Li, Kai Xu, Ruonan Zhou, Ming Lou, Yujie Du, Denis Music, Keke Chang
-
2025 | Article in journal
Atomistic assessment of interfacial interaction potential in tungsten twist grain boundaries
Praveenkumar Hiremath, Solveig Melin, Pär A T Olsson
-
2024 | Article in journal
Magnitnaya struktura Fe5O6: teoretiko-gruppovoy analiz i DFT-raschet
V. S. Zhandun, N. V. Kazak, D. M. Vasiukov
-
2024 | Conference paper
Effect of Varying External Conditions on Internal Frost Damage in Concrete
Lang-Zi Chang, Katja Frid, Karin Lundgren
-
2024 | Article in journal
Investigating Elastic Deformation of Ordered Precipitates by Ab Initio-Informed Phase-Field Crystal Modeling
Jacob Holmberg-Kasa, Pär A T Olsson, Martin Fisk
-
2024 | Article in journal
Evaluation of δ-Phase ZrH 1.4 to ZrH 1.7 Thermal Neutron Scattering Laws Using Ab Initio Molecular Dynamics Simulations
Vedant Mehta, Rehn Daniel, Pär A T Olsson
-
2024 | Article in journal
A level set approach to modelling diffusional phase transformations under finite strains with application to the formation of Cu 6 Sn 5
Erik Jacobsson, Håkan Hallberg, Johan Hektor, Srinivasan Iyengar, Matti Ristinmaa
-
2024 | Article in journal
First-principles study on thermal expansion of W-Re sigma and chi phases
Anders Vesti, Denis Music, Pär A T Olsson
-
Research project
Hierarchical nanoengineered assemblies of titania (Hi-NEAT)
riya.thomas@mau.se -
Research project
Impurity-induced embrittlement and fracture of irradiated liquid metal plasma-facing fusion reactor materials
par.olsson@mau.se -
Research project
Amorphous TiNiSn for efficient green energy generation
denis.music@mau.se -
Research project
High translucent zirconium dioxide ceramic materials: Studies on how processing factors affect material properties and performance
camilla.johansson@mau.se
Doctoral studies
Malmö universitet har forskarutbildning i forskarutbildningsämnet tillämpad fysik.
Applied Physics refers to the parts of physics that are relevant to technical applications and natural phenomena. For the education in Malmö, this includes materials science, atomic- and astrophysics and synchrotron light physics with applications.
Contact for doctoral studies in applied physics: Henrik Hartman